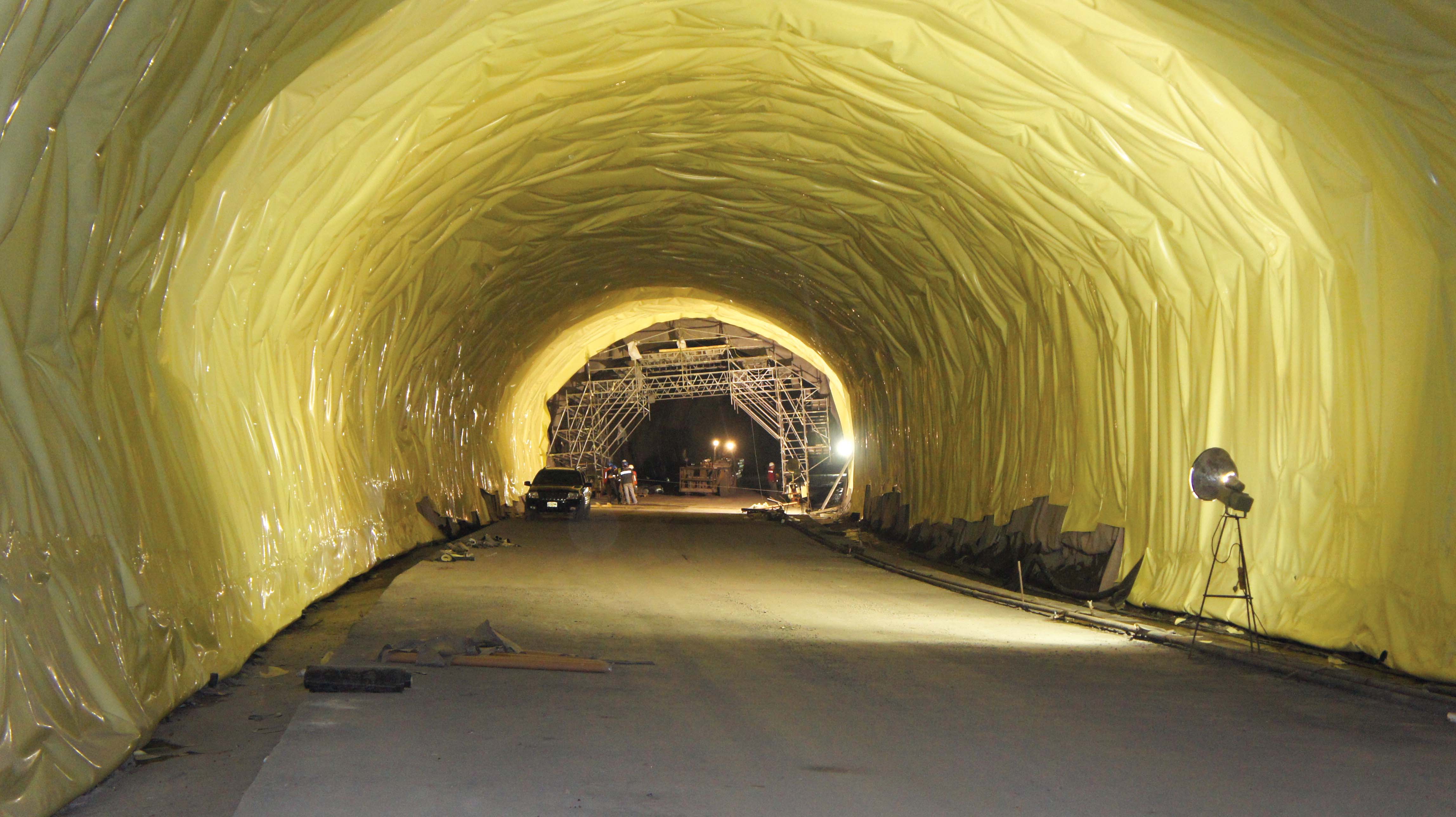
A new highway in Mexico is connecting Necaxa with Tihuatlan and the project features challenging terrain - Mauro Nogarin reports
In Mexico a landmark highway project is now close to completion, having set a number of records for Latin America. The Nueva Necaxa-Tihuatlan highway follows a route 85km long, with work on the project being carried out by the contractors
This stretch of highway is expected to open to traffic in the latter part of 2014 and once the final link is complete, the route will provide the shortest route between Mexico City and the coast. The highway is important for tourism, commerce and industry as Tuxpan is a tourist area with beaches as well as a busy port and lies near to the important oilfields of Poza Rica.
The new highway also links up with the existing highway between Tampico-Matamoros-Reynosa and the US border at Brownsville, Texas. Mexico City is the single most important economic area within Latin America and the new highway’s connection will help to further develop trade between Mexico and the US.
Work on the Nueva Necaxa-Tihuatlan highway has been divided into two sections, with TC1 running between Nueva Necaxa and Avila Camacho, a total distance of 36.6km.
Featuring four lanes, this 36km stretch is 21m wide and was built by FCC in conjunction with Mexican company ICA, requiring some 18.5 million m3 of material to be shifted during the construction. This stretch of highway has been particularly challenging to construct as it traverses the eastern Sierra Madre Mountains, in an area of great orographic difficulty, which required the construction of six tunnels and 12 viaducts.
The six tunnels stretch for a combined distance of 8.05km, while the 12 bridges span a total distance of 3.165km. Of the bridges, the structure over the San Marcos River is particularly impressive as it spans a distance of 850m and at its centre is 225m high, making it the second highest in the world. Only the Millau Viaduct in France has higher bridge supports.
The section of the Nueva Necaxa-Tihuatlan highway running between Avila Camacho and Tihuatlan is 48.1km long and is a 12m wide toll road featuring two lanes. It runs between the 178+500 and 225+866 distance markers and has been constructed through the rolling foothills of the eastern Sierra Madre Mountains, with 6.5 million m3 of material having been moved during its construction. This stretch is designed for vehicle speeds of between 90-110km/h and has four on/off ramps at the cities of Avila Camacho, Mecapalapa, Venustiano Carranza and Tihuatlan.
The highway between Nueva Necaxa and Tihuatlan was built at a cost of US$625 million, of which $339 was spent on the first section between Nueva Necaxa and Avila Camacho, with $190 million on the second part between Avila Camacho and Tihuatlan. When the Nueva Necaxa-Tihuatlan stretch of highway opens for traffic, it will reduce journey times by up to 2 hours, cutting the overall journey time between Mexico City and Tuxpan to around 3¼ hours.
The Sierra Madre range features a number of high mountains with very deep ravines in-between them. Altitudes above sea level range from 700 - 2,160m. The highest mountains are located to the north-west of Huachinango, near Tlalcoyunga, then dropping in height as they approach the coast of the Gulf of Mexico.
The construction area is also located in the Central Eastern Hydrological Basin, which is itself located in the Tuxpan-Nautla Hydrological Area, which includes a large number of permanent streams, the main water courses being the Necaxa and San Marcos Rivers.
San Marcos Bridge
The San Marcos Bridge is one of the most important in Mexico, because it holds two records. At 208m, the main support is the highest in the country, while its base is also the largest in Mexico. Measuring 34 x 34m, the base of the column features a truncated pyramid shape and is 7.5m high. The San Marcos Bridge features a novel pre-stressed concrete beam design and has a total length of 810m with seven spans, the longest of which are 180m long. The spans measure 45m, 90m, 180m, 180m, 180m, 90m and 45m respectively, while the bridge has a single deck carrying both carriageways.
San Marcos Bridge
Length:
810m
Width:
18.7m
Base of the column:
34 x 34m
Concrete volume of the base:
6,011m³
Height of column P4
208m
Geophysical studies were crucial to the construction of the bridge with the first part of this work being carried out using seismic tests. In all 16 seismic refractions were taken, each 60m in length and separated by geophones of 5m, with five localised sources. The data was then processed using Seisimager2D software, revealing that the area features four main layers. The surface covering comprises residual clay soil and rock fragments to a thickness of 4m, while in the middle of the channel the San Marcos River has a 2m thick layer consisting of sands and gravels. Underlying strata is of fractured rock and limestone, with inter-bedded shale, with an average thickness of 10m. Meanwhile at a depth of 13-15m there is semi-fractured limestone.
To provide a firm base for column P4 a direct footing was used onto a base measuring 32.5 x 21m and 5m deep. To build the bridge, it was necessary to divert the river during construction by building a temporary dam constructed of 45,000m3 of rocky material. To then construct the base it was necessary to use 64 piles, each 1.5m in diameter with variable lengths depending on the depth of the bedrock.
These varied in length from 9-25m, taking into account that the penetration of the piles into the bedrock was more than 2.5m. It was necessary to drill into the bedrock using a Bauer BG 28 and BG 24 drill, which allowed the installation of 1.5 piles/day on average. Once the holes were drilled, they were washed out to ensure that the debris was removed and would not contaminate the reinforced concrete piles.
The total steel rebar used for the 64 piles weighed in at 150tonnes, while to hoist the piles into place a Link Belt 118 crane was employed. For casting the piles, a total of 6,011m³ of concrete was used and this also featured a special fluidisation additive, which allowed the concrete to be placed without having to vibrate the mixture. Meanwhile the construction of column P4 was carried out with the help of a self-climbing formwork which used climbing equipment and advanced concrete pumping technology to allow for a higher yield.
To allow the movement of vehicles and equipment over the river, a 60m-span temporary bailey-type truss bridge was erected, while a parallel suspension footbridge was also constructed.
One of the biggest challenges facing the construction of the bridge columns was the placement of reinforcing steel. As a result equipment was located on site that was able to handle the threading of the steel, also helping to minimise steel wastage and meet the required production goals.
It was decided to use mechanical connections with threaded screw connectors and reinforced floor panels, which were pre-assembled on the floor in 10m sections, so that they did not exceed the crane’s lifting capacity. This method of placement also boosted safety, because it reduced the work required to place the pre-assembled panels and the time that the workers were exposed to high elevations. Meanwhile due to the difficulty of handling the formwork at heights of over 100m under difficult climatic conditions, it was decided to use a self-climbing system for the construction of column P4.
The self-climbing formwork consists of raising the panels hydraulically and with automated operation, which does not require support from the crane after being assembled and installed, so that the run times of the column are optimised and in turn increasing the required safety of personnel working on the elements.
Another major challenge was to carry large volumes of concrete to heights of over 61m to build the columns and the superstructure of the bridge itself. This was achieved by using a Putmeizter concrete pump with the ability to move material 360m vertically. The grade of concrete was also essential to the success of the entire superstructure and the best option was self-compacting material.
Huauchinango Tunnel
Despite the relatively short length of the Huauchinango tunnel, the construction work was tricky due to a number of factors. One issue was the location of oil pipelines as well as power transmission lines above the route of the tunnel. The work was further complicated by the soft soil conditions and another problem arose when exploratory drilling revealed the presence of a clayey, sandy silt material in the upper part of the slope.
This had a moisture content of 60-70%, and had a thickness of 5-10m. Due to the tricky conditions, the excavation of the tunnel was carried out using the New Austrian Tunnelling Method (NATM) and handled in two stages, first for the upper section and then for the lower section, with much of the excavation being carried out in basaltic volcanic rock.
The excavation of the tunnels modified the stress conditions of the soil and induced an inward movement of the excavation. To address deformation and prevent deterioration of the material, extensive support was required. The excavation was carried out with maximum advances of 1m, followed by immediate placement of support consisting of metal frames, shotcrete, anchors and drains. Only after these support measures were taken was it possible to continue pushing forward a further 1m advance and then placing subsequent supports. Umbrella drilling was carried out using 102mm diameter steel tubes.
Once the digging was completed for the upper half of the tunnel circumference, then the excavation of the bottom portion was able to commence using mechanical methods. The same process was then repeated on the lower section of the tunnel by inserting metal rods and layering concrete.
To provide the road structure, the fractured basaltic rock was used as the base with a layer of draining granular material topped by a 150mm granular base.
Huauchinango Tunnel
Twin tube tunnel with unidirectional
traffic flow for both bores
Design speed:
90km/h
Length:
right bore 105.45m; left bore 89m
General incline:
right bore 0.73%; left bore 0.96%
Entrance level (grade):
1217.45 (right tunnel)
and 1218.18 (left tunnel)
Exit level (Flush):
1216.68 (right tunnel)
and 1217.32 (left tunnel)
Width of roadway:
10m
Height of tunnel:
8.35m
Total width of tunnel:
13.6m
Area of excavation:
99.45m2 each
The waterproofing system planned for the Huauchinango tunnel includes a geotextile that partly protects the waterproofing membrane from irregularities, and also prevents water from draining through the support to the shotcrete. Another key part of the waterproofing system features lateral drains that collect the water gathered by the waterproofing system. These drains are made of 102mm diameter PVC pipes, with interceptors spaced every 10m connecting with side drainage sumps specifically for water infiltration.
Once the digging was completed for the upper half of the tunnel circumference, then the excavation of the bottom portion was able to commence using mechanical methods. The same process was then repeated on the lower section of the tunnel by inserting metal rods and layering concrete.
To provide the road structure, the fractured basaltic rock was used as the base with a layer of draining granular material topped by a 150mm granular base.
The waterproofing system planned for the Huauchinango tunnel includes a geotextile that partly protects the waterproofing membrane from irregularities, and also prevents water from draining through the support to the shotcrete.
Another key part of the waterproofing system features lateral drains that collect the water gathered by the waterproofing system. These drains are made of 102mm diameter PVC pipes, with interceptors spaced every 10m connecting with side drainage sumps specifically for water infiltration.